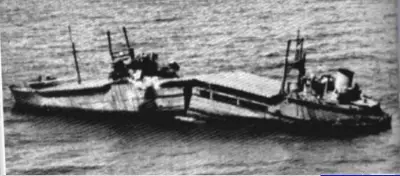
As was written, the distinction between brittleness and ductility isn’t readily apparent, especially because both ductility and brittle behavior are dependent not only on the material in question but also on the temperature (ductile-brittle transition) of the material. The effect of temperature on the nature of the fracture is of considerable importance. Many steels exhibit ductile fracture at elevated temperatures and brittle fracture at low temperatures. The temperature above which a material is ductile and below which it is brittle is known as the ductile–brittle transition temperature (DBTT), nil ductility temperature (NDT), or nil ductility transition temperature. This temperature is not precise, but varies according to prior mechanical and heat treatment and the nature and amounts of impurity elements. It can be determined by some form of drop-weight test (for example, the Charpy or Izod tests).
The ductile–brittle transition temperature (DBTT) is the temperature at which the fracture energy passes below a predetermined value (e.g. 40 J for a standard Charpy impact test). Ductility is an essential requirement for steels used in the construction of reactor components, such as the reactor vessel. Therefore, the DBTT is of significance in the operation of these vessels. In this case, the size of the grain determines the properties of the metal. For example, smaller grain size increases tensile strength, tends to increase ductility and results in a decrease in DBTT. Grain size is controlled by heat treatment in the specifications and manufacturing of reactor vessels. The DBTT can also be lowered by small additions of selected alloying elements such as nickel and manganese to low-carbon steels.
Typically, the low alloy reactor pressure vessel steels are ferritic steels that exhibit the classic ductile-to-brittle transition behaviour with decreasing temperature. This transitional temperature is of the highest importance during plant heatup.
Failure modes:
- Low toughness region: Main failure mode is the brittle fracture (transgranular cleavage). In brittle fracture, no apparent plastic deformation takes place before fracture. Cracks propagate rapidly.
- High toughness region: Main failure mode is the ductile fracture (shear fracture). In ductile fracture, extensive plastic deformation (necking) takes place before fracture. Ductile fracture is better than brittle fracture, because there is slow propagation and an absorption of a large amount energy before fracture.
In some materials, the transition is sharper than others and typically requires a temperature-sensitive deformation mechanism. For example, in materials with a body-centered cubic (bcc) lattice the DBTT is readily apparent, as the motion of screw dislocations is very temperature sensitive because the rearrangement of the dislocation core prior to slip requires thermal activation. This can be problematic for steels with a high ferrite content. This famously resulted in serious hull cracking in Liberty ships in colder waters during World War II, causing many sinkings. The vessels were constructed of a steel alloy that possessed adequate toughness according to room-temperature tensile tests. The brittle fractures occurred at relatively low ambient temperatures, at about 4°C (40°F), in the vicinity of the transition temperature of the alloy. It must be noted that low-strength FCC metals (e.g. copper alloys) and most HCP metals do not experience a ductile-to-brittle transition and retain tough also for lower temperatures. On the other hand, many high-strength metals (e.g. very high-strength steels) also do not experience a ductile-to-brittle transition, but, in this case, they remain very brittle.
DBTT can also be influenced by external factors such as neutron radiation, which leads to an increase in internal lattice defects and a corresponding decrease in ductility and increase in DBTT.
Irradiation Embrittlement
During the operation of a nuclear power plant, the material of the reactor pressure vessel and the material of other reactor internals are exposed to neutron radiation (especially to fast neutrons >0.5MeV), which results in localized embrittlement of the steel and welds in the area of the reactor core. This phenomenon, known as irradiation embrittlment, results in the steadily increase in DBTT. It is not likely that the DBTT will approach the normal operating temperature of the steel. However, there is a possibility that when the reactor is being shut down or during an abnormal cooldown, the temperature may fall below the DBTT value while the internal pressure is still high. Therefore nuclear regulators require that a reactor vessel material surveillance program be conducted in watercooled power reactors.
See also: Neutron Reflector
Irradiation embrittlement can lead to loss of fracture toughness. Typically, the low alloy reactor pressure vessel steels are ferritic steels that exhibit the classic ductile-to-brittle transition behaviour with decreasing temperature. This transitional temperature is of the highest importance during plant heatup.
Failure modes:
- Low toughness region: Main failure mode is the brittle fracture (transgranular cleavage). In brittle fracture, no apparent plastic deformation takes place before fracture. Cracks propagate rapidly.
- High toughness region: Main failure mode is the ductile fracture (shear fracture). In ductile fracture, extensive plastic deformation (necking) takes place before fracture. Ductile fracture is better than brittle fracture, because there is slow propagation and an absorption of a large amount energy before fracture.
Neutron irradiation tends to increase the temperature (ductile-to-brittle transition temperature) at which this transition occurs and tends to decrease the ductile toughness.
We hope, this article, Ductile–brittle Transition Temperature, helps you. If so, give us a like in the sidebar. Main purpose of this website is to help the public to learn some interesting and important information about materials and their properties.