Forms of Ionizing Radiation
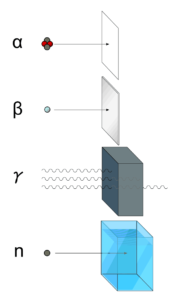
Ionizing radiation is categorized by the nature of the particles or electromagnetic waves that create the ionizing effect. These particles/waves have different ionization mechanisms, and may be grouped as:
- Directly ionizing. Charged particles (atomic nuclei, electrons, positrons, protons, muons, etc.) can ionize atoms directly by fundamental interaction through the Coulomb force if it carries sufficient kinetic energy. These particles must be moving at relativistic speeds to reach the required kinetic energy. Even photons (gamma rays and X-rays) can ionize atoms directly (despite they are electrically neutral) through the Photoelectric effect and the Compton effect, but secondary (indirect) ionization is much more significant.
- Alpha radiation. Alpha radiation consist of alpha particles at high energy/speed. The production of alpha particles is termed alpha decay. Alpha particles consist of two protons and two neutrons bound together into a particle identical to a helium nucleus. Alpha particles are relatively large and carry a double positive charge. They are not very penetrating and a piece of paper can stop them. They travel only a few centimeters but deposit all their energies along their short paths.
- Beta radiation. Beta radiation consist of free electrons or positrons at relativistic speeds. Beta particles (electrons) are much smaller than alpha particles. They carry a single negative charge. They are more penetrating than alpha particles, but thin aluminum metal can stop them. They can travel several meters but deposit less energy at any one point along their paths than alpha particles.
- Indirectly ionizing. Indirect ionizing radiation is electrically neutral particles and therefore does not interact strongly with matter. The bulk of the ionization effects are due to secondary ionizations.
- Photon radiation (Gamma rays or X-rays). Photon radiation consist of high energy photons. These photons are particles/waves (Wave-Particle Duality) without rest mass or electrical charge. They can travel 10 meters or more in air. This is a long distance compared to alpha or beta particles. However, gamma rays deposit less energy along their paths. Lead, water, and concrete stop gamma radiation. Photons (gamma rays and X-rays) can ionize atoms directly through the Photoelectric effect and the Compton effect, where the relatively energetic electron is produced. The secondary electron will go on to produce multiple ionization events, therefore the secondary (indirect) ionization is much more significant.
- Neutron radiation. Neutron radiation consist of free neutrons at any energies/speeds. Neutrons can be emitted by nuclear fission or by the decay of some radioactive atoms. Neutrons have zero electrical charge and cannot directly cause ionization. Neutrons ionize matter only indirectly. For example, when neutrons strike the hydrogen nuclei, proton radiation (fast protons) results. Neutrons can range from high speed, high energy particles to low speed, low energy particles (called thermal neutrons). Neutrons can travel hundreds of feet in air without any interaction.
Beta Radiation
Beta radiation consist of free electrons or positrons at relativistic speeds. These particles are known as the beta particles. Beta particles are high-energy, high-speed electrons or positrons emitted by certain fission fragments or by certain primordial radioactive nuclei such as potassium-40. The beta particles are a form of ionizing radiation also known as beta rays. The production of beta particles is termed beta decay. There are two forms of beta decay, the electron decay (β− decay) and the positron decay (β+ decay). In a nuclear reactor occurs especially the β− decay, because the common feature of the fission products is an excess of neutrons (see Nuclear Stability). An unstable fission fragment with the excess of neutrons undergoes β− decay, where the neutron is converted into a proton, an electron, and an electron antineutrino.
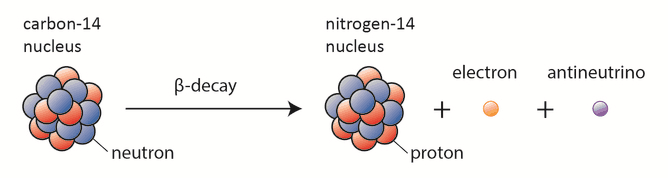
Characteristics of Beta Radiation
Key characteristics of beta radiation are summarized in following points:
- Beta particles are energetic electrons, they are relatively light and carry a single negative charge.
- Their mass is equal to the mass of the orbital electrons with which they are interacting and unlike the alpha particle a much larger fraction of its kinetic energy can be lost in a single interaction.
- Their path is not so straightforward. The beta particles follow a very zig-zag path through absorbing material. This resulting path of particle is longer than the linear penetration (range) into the material.
- Since they have very low mass, beta particles reach mostly relativistic energies.
- Beta particles also differ from other heavy charged particles in the fraction of energy lost by radiative process known as the bremsstrahlung. Therefore for high energy beta radiation shielding dense materials are inappropriate.
- When the beta particle moves faster than the speed of light (phase velocity) in the material it generates a shock wave of electromagnetic radiation known as the Cherenkov radiation.
- The beta emission has the continuous spectrum.
- A 1 MeV beta particle can travel approximately 3.5 meters in air.
- Due to the presence of the bremsstrahlung low atomic number (Z) materials are appropriate as beta particle shields.
Shielding of Beta Radiation – Electrons
Beta radiation ionizes matter weaker than alpha radiation. On the other hand the ranges of beta particles are longer and depends strongly on initial kinetic energy of particle. Some have enough energy to be of concern regarding external exposure. A 1 MeV beta particle can travel approximately 3.5 meters in air. Such beta particles can penetrate into the body and deposit dose to internal structures near the surface. Therefore greater shielding than in case of alpha radiation is required.
Materials with low atomic number Z are appropriate as beta particle shields. With high Z materials the bremsstrahlung (secondary radiation – X-rays) is associated. This radiation is created during slowing down of beta particles while they travel in a very dense medium. Heavy clothing, thick cardboard or thin aluminium plate will provide protection from beta radiation and prevents of production of the bremsstrahlung.
See also more theory: Interaction of Beta Radiation with Matter
See also calculator: Beta activity to dose rate
Shielding of Beta Radiation – Positrons
The coulomb forces that constitute the major mechanism of energy loss for electrons are present for either positive or negative charge on the particle and constitute the major mechanism of energy loss also for positrons. Whatever the interaction involves a repulsive or attractive force between the incident particle and orbital electron (or atomic nucleus), the impulse and energy transfer for particles of equal mass are about the same. Therefore positrons interact similarly with matter when they are energetic. The track of positrons in material is similar to the track of electrons. Even their specific energy loss and range are about the same for equal initial energies.
At the end of their path, positrons differ significantly from electrons. When a positron (antimatter particle) comes to rest, it interacts with an electron (matter particle), resulting in the annihilation of the both particles and the complete conversion of their rest mass to pure energy (according to the E=mc2 formula) in the form of two oppositely directed 0.511 MeV gamma rays (photons).
Therefore any positron shield have to include also a gamma ray shield. In order to minimize the bremsstrahlung a multi-layered radiation shield is appropriate. Material for the first layer must fulfill the requirements for negative beta radiation shielding. First layer of such shield may be for example a thin aluminium plate (to shield positrons), while the second layer of such shield may be a dense material such as lead or depleted uranium.
See also: Shielding of Gamma Radiation
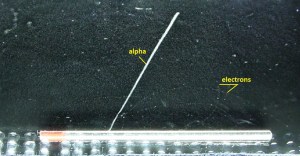
Source: wikipedia.org
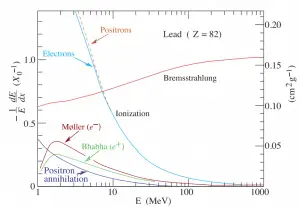
function of electron or positron energy. Source: http://pdg.lbl.gov/
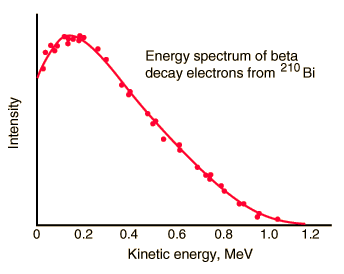
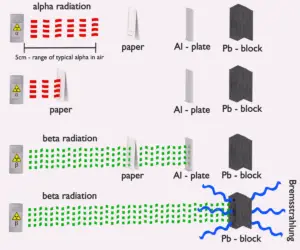
We hope, this article, Beta Radiation, helps you. If so, give us a like in the sidebar. Main purpose of this website is to help the public to learn some interesting and important information about materials and their properties.