Charged Particle Ejection
Charged particle reactions are usually associated with formation of a compound nucleus, which is excited to a high energy level, that such compound nucleus can eject a new charged particle while the incident neutron remains in the nucleus. After the new particle is ejected, the remaining nucleus is completely changed, but may or may not exist in an excited state depending upon the mass-energy balance of the reaction. This type of reaction is more common for charged particles as incident particles (such as alpha particles, protons, and so on).
The case of neutron-induced charged particle reactions is not so common, but there are some neutron-induced charged particle reactions, that are of importance in the reactivity control and also in the detection of neutrons.
The most important charged particle reactions in nuclear reactor physics are reactions of thermal neutrons with boron nuclei (rather with 10B nuclei). In nuclear industry boron is commonly used as a neutron absorber and a neutron converter (in neutron radiation detectors) due to its high neutron cross-section of isotope 10B along entire neutron energy spectrum. Its (n,alpha) reaction cross-section for thermal neutrons is about 3840 barns (for 0.025 eV neutron). Examples of neutron-induced charged particle reactions are shown below:
See also: Application of boron based materials
See also: Detection of neutrons
σ
Examples of (n,alpha) reactions
10B(n,alpha)7Li
This reaction is the most important (n,alpha) reaction of isotope 10B with thermal neutrons. This reaction can be used as in the case of neutron absorbers (chemical shim, burnable absorbers or control rods), and in the case of neutron converters (neutron detectors), because its cross-section is very high and produces energetic alpha particles.
6Li(n,α)3H
This is a reaction allowing detection of neutrons. The reaction cross-section for thermal neutrons is σ = 925 barns and the natural lithium has abundance of 6Li 7,4%.
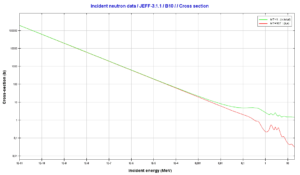
Source: JANIS (Java-based Nuclear Data Information Software); The JEFF-3.1.1 Nuclear Data Library
Source: JANIS (Java-based Nuclear Data Information Software); The JEFF-3.1.1 Nuclear Data Library
Examples of (n,p) reactions
3He(n,p)3H
This is a reaction allowing detection of neutrons. The reaction cross-section for thermal neutrons is σ = 5350 barns and the natural helium has abundance of isotope 3He 0.014%.
14N (n,p) 14C
This nuclear reaction continually take place especially in the earth’s atmosphere, forming equilibrium amounts of the radionuclide 14C. In nuclear power plants, it is important especially from radiation protection point of view. The reaction is responsible for most of the radiation dose delivered to the human body by thermal neutrons. The nitrogen atoms are contained in proteins, therefore if the human body is exposed to thermal neutrons, then these thermal neutrons may be absorbed by 14N, causing a proton emission. Protons are directly ionizing particles and deposit their energy over a very short distance in the body tissue.
Examples of (n,…) reactions
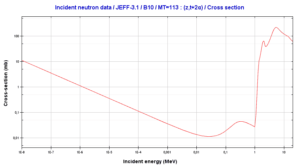
10B(n,T + 2*alpha)
This threshold reaction of fast neutron with an isotope 10B is the main way, how radioactive tritium in primary circuit of all PWRs is generated. 10B is the principal source of radioactive tritium in primary circuit of all PWRs (which use boric acid as a chemical shim). Note that, this reaction occurs very rarely in comparison with the most common (n,alpha) reaction of isotope 10B with thermal neutrons.
There are more reactions with neutrons, which can rarely lead to formation of radioactive tritium, for example:
10B(n,alpha)7Li + 7Li(n,n+alpha)3H – threshold reaction (~3 MeV).
We hope, this article, Charged Particle Ejection, helps you. If so, give us a like in the sidebar. Main purpose of this website is to help the public to learn some interesting and important information about materials and their properties.